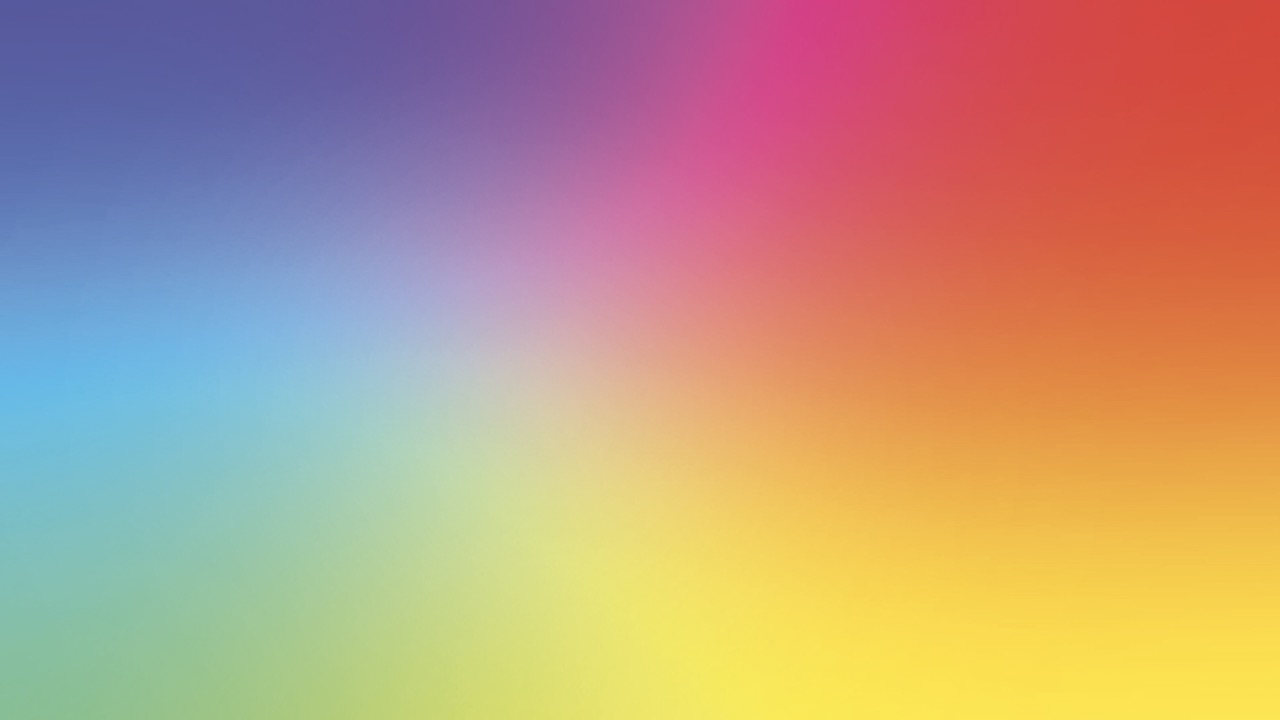
Lighting special: Bright ideas
Posted on Feb 4, 2025 by Admin
The quest for colour-perfect, user-friendly lighting continues – and 2025 might just deliver, says Phil Rhodes
Making a candle requires some wax and a piece of string. Making LED lights requires a semiconductor foundry and an electronics manufacturing plant. There’s a reason for all that tech: it gives us options. Historical lighting had just one control and rarely strayed from its intended colour. Recapturing that simplicity has been a design goal since the advent of LED lights, and 2025 might see a bit of progress in that direction.
The simplicity of classic lighting – tungsten light bulbs, sunlight and candles – lies in the fact that they emit light by glowing due to heat. LEDs, along with fluorescent tubes and HMIs, don’t glow because they’re hot, yet an immense effort has gone into making them mimic this behaviour. Even so, the challenge goes beyond producing white light. We want lighting with the colour quality of an open flame, paired with high efficiency, ease of use and all the modern conveniences in one device.
Understanding this starts with knowing how LEDs produce white light. We might try combining red, green and blue to create a light that looks superficially white when directed at a grey card. Shine it on something orange, however, and the limitation becomes apparent: the orange reflects wavelengths of orange light, which sit between red and green on the spectrum. It might reflect some of the light from the red LEDs, but it probably doesn’t reflect much green, so we get a neon-red blood orange.
It will also make human beings look blotchy and florid, which is why we don’t make movie lights out of RGB clusters (repurposed disco lights and virtual production video walls notwithstanding). The solution which changed the world was to coat a blue LED with a yellow-emitting fluorescent phosphor. The two combine to look approximately like something hot enough to glow.
In early designs, that might have been very approximate. Many late nights in R&D labs around the world yielded enough improvement for us to rely on it for everything, from flashlights to film sets.
It’s an imperfect solution, often lacking teal and deep blue. A phosphor can only make light redder. It can turn blue into red, orange or green, but it can’t turn blue into a deeper blue. White-emitting LEDs have long struggled with a lack of deep blue-violet colours, which affects how white fabrics and even skin are rendered. Overcoming this challenge required low-level engineering. While blue LEDs were tricky to develop, violet was even tougher and has only recently become practical for high-power lighting.
Even before that, the demand for features had already led designers to combine phosphor-converted white LEDs with other types. Take a tungsten-balanced phosphor white, add a blue emitter, and fading in that blue emitter moves the light towards daylight. Add red and green, and we can achieve any colour we like, including plus- or minus-green adjustments.
Big-name manufacturers have built essentially that design, but the further from the original white we go, the more it relies on narrow-spectrum colour emitters – and the worse the colour quality gets. Some early lights with variable colour temperature had noticeably poorer colour rendering in daylight than tungsten.
More advanced designs incorporate two different white emitters. The latest might not include a white emitter at all, instead relying on other colours to build a high-quality white. This invariably involves phosphor conversion to colours called cyan, mint, teal, lime, amber or orange. Similar to the phosphor white emitters, these colours offer a broader spectrum. The result is a smoother white spectrum and fewer caveats.
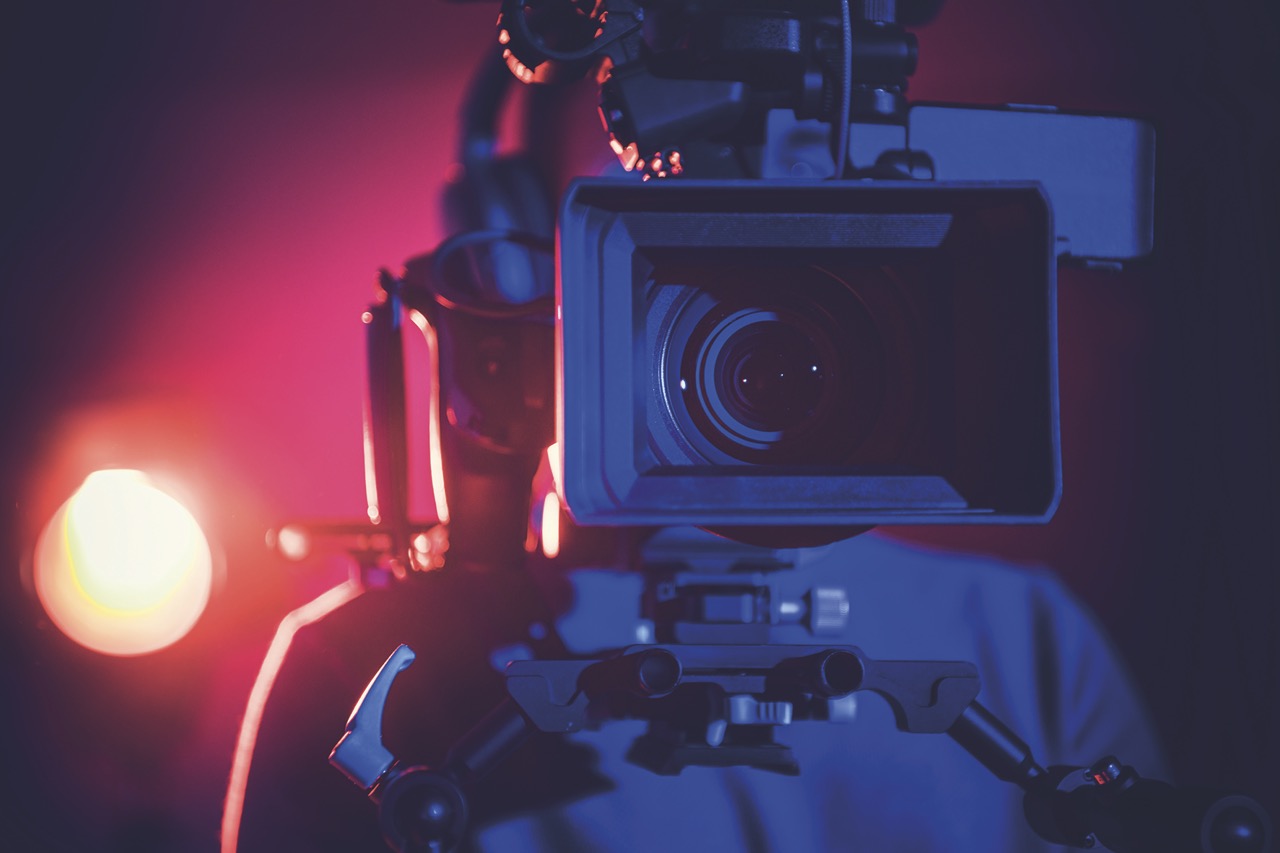
Measuring colour quality
This has given us the most capable lighting we’ve ever known. However, it heavily applies technology to something we mostly prefer to keep simple. Daylight should look like daylight, tungsten should appear as tungsten, and when illuminating brightly coloured objects or people, the results should be free of unpleasant surprises.
It’s natural, perhaps, to look for a straightforward and numeric score which might express how well a light performs, but it’s difficult (actually, it’s impossible) to boil a spectrum of light down to a single number. Even interacting with the user interface in a modern light might mean knowing how one or more colour charts work.
One of the simplest ways to evaluate light quality is by shining it on a series of colours and measuring how well each is illuminated. Averaging the results across many colours provides an overall indicator of a white light’s overall colour quality. For TLCI, the colour patches are taken from the famous Macbeth chart, which works reasonably well because the chart includes some saturated shades of the primary and secondary colours. If the light lacks cyan, for instance, the cyan chip will look dull and the score will reflect that deficiency.
The counterexample is CRI, which uses only a fairly pale, desaturated set of colours. Even mediocre lights can illuminate test patches which reflect a wide range of colours, masking some underlying problems. As a result, CRI is far less sensitive than TLCI. While TLCI is TV-orientated and no set of colour patches can cover every possibility, it’s rare for a light to measure a high TLCI and still create serious problems.
More sensitive measurements include the Spectral Similarity Index (SSI), which compares any two light sources. It is often used to evaluate how closely an artificial light matches sunlight, which can be expressed as SSI[CIE D55] for a standard 5500K daylight source. Colorimeters typically make measurements to any of those standards – but still, none of these methods provide us with a definitive yes-or-no answer.
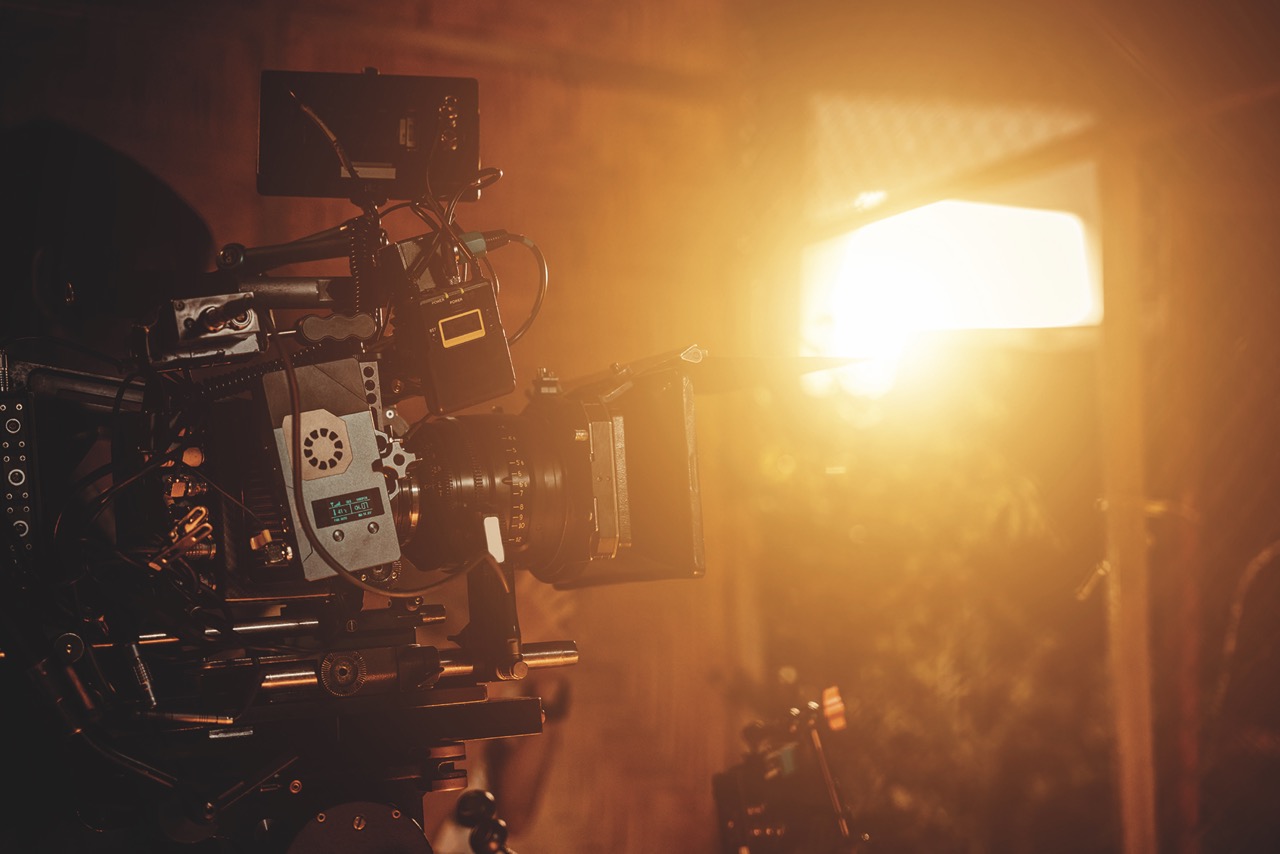
Controls and matching
It’s rare, though, to find a modern LED that struggles to create a reasonable white. The bigger issue is finding two which create the same white. Modern LEDs are also the first movie lights that can mix colours to taste, and if we’ve picked a shade of blue-green for our moonlight, it would be great if all of our lights matched when we programme them with the same numbers.
Humans are terrible at remembering what colour something is – even over minutes – but the human eye (or the camera) is exquisitely sensitive to a comparison of two lights side by side. Both are more sensitive to comparisons than a colorimeter anyone might carry around. So, matching two lights with high accuracy might take some manual adjustment – albeit from the comfort of a phone app as opposed to up a ladder with some gels.
Where things might differ is in how the controls actually work. Most people have at least seen a CIE 1931 chart and understand that it represents all visible colours. Any point on that chart represents a colour as seen by a human or (ideally, but not invariably) a camera. Set the same XY coordinates on two lights, and they should be identical in colour. Between two little-used lights employing the same underlying emitter technology from a single manufacturer, that can work fairly well.
Moving a crosshair around a CIE chart by turning controls is not always intuitive. Not all lights implement it, and the alternatives may not be well standardised. Some, for instance, let users set a hue, saturation and brightness. Traditionally, zero degrees of hue means red, although there is no universal agreement on the shade of red, what 50% saturation means or what brightness means for different colours; yellow might look brighter than blue at the same number.
There are dozens of other approaches, and the relative inaccessibility of all that is what provokes lights to let us choose a virtual light source – HMI, tungsten and so on – and then add a virtual gel. The accuracy of that simulation varies, particularly where deep blues (like LEE Filters’ #181 Congo Blue) need that new deep-violet emitter technology, but it’s familiar. If the near future lets us do that with more confidence, nobody will object.
That approach, though, encapsulates something of a recognition that LEDs will always be complicated compared to those legacy lights, which had just one switch to throw. Given the current enthusiasm for classical techniques in filmmaking, it’s no surprise we all want LEDs which combine the viceless predictability of old light bulbs with all the options of modern designs. Still, if deep blue was among the last unattained peaks of sheer capability in LED lighting, the next few years seem likely to be about control, accuracy and matching.
It’s always tricky to recognise an epoch as it’s happening. LED technology in general has certainly represented one, but whether we’re about to encounter another as the field slides into maturity remains to be seen.
Read more from our Lighting Special with this article about shooting for day and night.
This story appears in the January 2025 issue of Definition